Amazing investment in LHCb from UKRI
15 June 2022
Today is a good-news day by any measure. UKRI has announced genuinely transformational funding for LHCb 2030+, the project that will enable the UK's central role in the next major upgrade of the LHCb experiment at CERN. The investment of almost £50M over a ten-year period is a resounding endorsement at the highest level of the science and societal impact of our work.
Today we are announcing £481 million over the next three years in a portfolio of investments in UK’s research and innovation infrastructure.
— UK Research and Innovation (@UKRI_News) June 15, 2022
Learn more about the projects: https://t.co/6SBIilG5nr pic.twitter.com/m8uNs7hFiX
First LHCb measurement of W boson mass
17 January 2022
The LHCb collaboration has recently published in the Journal of High Energy Physics their first measurement of the mass of the W boson. Knowing the masses of the fundamental Z0, W± and Higgs bosons, together with the mass of the top quark, is vital to understanding the theory that underpins all of particle physics, the Standard Model (SM). The SM tells us how these masses should be related to each other, so measuring them to incredibly high precision at the Large Hadron Collider (LHC) tests how well the SM actually describes the world we live in. A poor agreement would indicate that the SM does not describe fundamental interactions, and could give hints of the existence of new particles or new symmetries in the universe.
The W boson is an unstable particle that is responsible for the radioactive β decay of atomic nuclei. The existence of the W boson was postulated in the 1960s by Sheldon Glashow, Abdus Salam and Steven Weinberg with the unification of the theories describing electromagnetic and weak-nuclear interactions. It was first observed twenty years later, in 1983, at CERN's Super Proton Synchrotron (SPS) collider.
This state-of-the-art LHCb measurement has been carried out by scientists from the University of Warwick, Imperial College London, the University of Liverpool and the University of Oxford. The analysis reports a value of mW = 80354 ± 23 (stat.) ± 10(exp.) ± 17 (theory) ± 9(pdf) MeV, obtained by processing proton-proton collision data collected in 2016. The result is in agreement with the SM and with previous measurements. UK scientists have led LHCb measurements of electroweak boson cross-sections over the last ten years, leading to significant improvements in our knowledge of the proton's internal structure. The figure on the left shows the current status of all global measurements of the W-boson mass, including this latest measurement, highlighted in orange.
This high-precision measurement is performed at LHCb using the transverse momentum of the outgoing lepton, ℓ, from the W→ℓν decay, which the detector measures with excellent precision. To further increase the accuracy of the measurement, a careful treatment of the detector calibration and the alignment of the subdetectors of LHCb is applied using specialised algorithms. The abundance of "standard candles" such as J/ψ, Υ(1S) and Z0 particles decaying in the LHCb acceptance provides a variety of samples to use as control modes in order to calibrate the measurement.
The W→μν decay is chosen to measure mW in the LHCb analysis, since it provides the cleanest signal with which to perform the measurement. Precise knowledge of the production rate of signal and background candidates in LHC collisions, together with an accurate description of the detector resolution, allows us to determine the shapes of the transverse momentum (shown in the figure below) for each of the different processes contributing to the observed spectrum. By considering different mass hypotheses for the W boson, a fit to the shapes of the signal (blue) and background candidates (orange, green, red and pink) to the data (black points) is used to infer the value of the W boson mass.
We have tested the inner workings of our universe, achieving incredible precision as we explore electroweak physics.Miguel Ramos Pernas, a post-doctoral researcher on the project from the University of Warwick, added
I'm excited both for this measurement, and for where our research goes next - we're only just beginning here.
LHCb have already started on the next challenge: to further improve the measurement by using three times more data, collected in 2017 and 2018. The goal of the current generation of LHC experiments is to provide a precise, combined measurement of the W boson mass close to the precision of the SM prediction, of around 7 MeV. This is expected to be possible after 2030, when the LHCb experiment will have collected a data sample some 25 times larger.
Stronger hints of a violation of lepton universality?
23 March 2021: 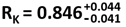
Today at the Rencontres de Moriond EW conference and CERN seminar, the LHCb Collaboration presented an updated measurement of the ratio RK. This tests an important principle of the Standard Model of particle physics known as "lepton universality", which says the three charged leptons (electrons, muons and taus) behave identically, apart from effects due to their different masses. An LHCb paper submitted for publication today presents evidence suggesting that lepton universality may be violated in beauty-quark decays, with a statistical significance of 3.1 σ (standard deviations).
Evidence is the term often used in the community when a measurement has a significance of more than three σ ; observation is typically reserved for results with more than five σ.
The ratio RK describes how often a B+ meson decays to a charged kaon and either a positive and a negatively charge muon (K+μ+μ-) or a positron-electron pair (K+e+e-). These decays are extremely rare, occurring at a rate of only one in two million B+ meson decays. The decays involve the transformation of a beauty quark into a strange quark (b→s), a process that is highly suppressed in the Standard Model and can be affected by the existence of new particles, which could have masses too high to be produced directly even at the Large Hadron Collider.
The analysis is performed in the range 1.1<q2<6.0 GeV2, where q2 is the invariant mass of the μ+μ- or e+e- pair. The value of RK is measured to be 0.846+0.044-0.041, and is shown in the figure to the left as a black point with error bars. This differs from the Standard Model prediction by 3.1σ, is the most precise measurement to date; it is consistent with and supersedes previous LHCb RK results, also shown on the plot as grey points with error bars. Measurements by the BaBar and Belle collaborations are also shown.
To reduce the influence of experimental factors, a double ratio method was used, measuring RK divided by another ratio, rJ/ψ. The rJ/ψ is defined as the ratio of the probability that a B+ decays to J/ψK+ (where J/ψ →μ+μ-) with the probability that a B+ decays to J/ψK+ (where J/ψ →e+e-). Although the true value of the rJ/ψ ratio is predicted to be very close to 1, it may also be affected by experimental factors that have a similar effect on RK. This is very helpful, because if there are any remaining effects that have not already been accounted for by exhaustive studies and corrections in measuring RK, they are expected to cancel in the double ratio between RK and rJ/ψ. In addition, as a J/ψ meson decays into μ+μ- and e+e- pairs respect lepton universality at the 0.4% level, a measurement of the single ratio, rJ/ψ makes an excellent "standalone" cross-check: it does not benefit from the double ratio's cancellation of systematic effects and therefore is a sensitive and rigorous test of the methods used to determine the efficiencies. The value of rJ/ψ is found to be 0.981±0.020, consistent with 1. The four figures on the right show the measured invariant mass distributions of B+ candidates for the four decay modes used in the double-ratio measurement, each with a clear accumulation of events around the B+ meson mass.
The improved precision makes this new result exciting, as it edges the statistical significance above the threshold for evidence that a key feature of the Standard Model of particle physics looks less certain. In the near future, LHCb will report results from the decays of other beauty particles and related measurements, adding more information to another tantalising puzzle that is emerging from LHC data.
Physicists from the Universities of Bristol, Cambridge and Imperial College London led the analysis of the data to produce this result; see related article in The Conversation - and an alternative take on why are are "cautiously excited" by the new result:
Strange and beautiful violations of symmetry
6 October 2020
The LHCb Collaboration has today presented two new analyses that uncover previously unseen properties of the strange and beautiful meson, which is a bound state made of one beauty b-quark and one strange s-quark.
One of the fundamental questions scientists at LHCb are trying to answer is: Why does the universe have such a large imbalance between matter and antimatter when they should have been created in equal amounts? Intimately related to this question is the topic of CP (Charge-Parity) violation: a violation of the symmetry between matter and antimatter. CP violation could explain why the universe is matter dominated, however the amount of CP violation we see in particle physics experiments is about a factor of 10 billion too small to account for cosmological observations. New results from LHCb, analysing rare B0s meson decays to two kaons, B0s→K+K−, and also to two pions, a kaon and a meson (a bound state of a strange and charm quark) B0s→D−sK+π+π−, help to shed further light on this mystery.
Due to the remarkable properties of quantum mechanics, the B0s meson rapidly oscillates, about 3 million million times per second, to its antiparticle (the B0s meson) and back again as it travels through space. This oscillation provides two different paths for a decay: either directly into the decay products or via an oscillation to its antiparticle, and then a subsequent decay. If the matter-antimatter symmetry were exact then the interference between these two paths would be equal for both particle and antiparticle. However, new results of B0s meson decays to two kaons (B0s→K+K−) presented today at a CERN seminar, have found a difference or asymmetry between these rates which varies as a function of time, shown in the upper figure, as the B0s oscillates back and forth to its antiparticle. This behaviour has also been confirmed by analysis of the meson in its decay to D−sK+π+π−, shown in the lower figure. These two figures show the difference between the particle and antiparticle rates as a function of decay time that without CP-violation would cancel giving a flat line at zero. In the lower figure it can be seen that the mixing asymmetries for the CP conjugate final states D−sK+π+π−and D+sK−π−π+are shifted with respect to each other which indicates a possible additional source of CP violation induced in the oscillations themselves.
The asymmetries in both of these decay channels depend on the same fundamental parameters but any differences between them, which are not seen at the current sensitivity, would be a very clear sign of New Physics Beyond the Standard Model.
Lars Eklund, of University of Glasgow and Uppsala University, who worked on the B0s→K+K− analysis said
This is the first unambiguous observation of a time-dependent asymmetry between matter and antimatter in B0s mesons, which is a milestone in the study of these phenomena. The Standard Model of Particle Physics predicts this asymmetry and measuring its size provides input to determining some of the fundamental parameters of the Standard Model.Eva Gersabeck, of Manchester University, who worked on the B0s→D−sK+π+π− analysis commented
Finalising this measurement is a very exciting moment, we have been working on it for a very long time. Only the fantastic resolution of the LHCb experiment allows for disentangling the rapid B0s oscillations and this measurement can not be done at any other experiment.
You can read more on LHCb’s public page.
Charming and strange: more exotic hadrons
11 August 2020
Today, at a CERN seminar, the LHCb collaboration announced the discovery of an exotic structure, hidden in B+→D+D−K+ decays. The exotic structure, seen at a mass of 2.9 GeV/c2 in the mass spectrum of the D−K+ pair, could be interpreted as the first discovery of an open-flavour tetraquark, or rather two, each having a different spin.
Hadrons are bound states of collections of quarks and antiquarks, held together by the strong interaction. In the decades following the development of the quark model, proposed independently by Murray Gell-Mann and George Zweig in 1964, two types of hadrons were seen. These are baryons, which are composed of three quarks (and antibaryons, with three antiquarks), and mesons, composed of a quark and an antiquark; collectively, these are referred to as conventional hadrons. But in recent years an increasing number of exotic hadrons have been observed that have minimal quark content of either two quarks and two antiquarks ("tetraquarks") or four quarks and one antiquark ("pentaquarks"), and that hence do not fit into this scheme. This raises questions about how precisely these exotic hadrons are bound together, and provides the exciting possibility of learning more about the behaviour of the strong interaction.
Until now all discovered exotic hadrons contained either a charm (c) quark and an anticharm (c) antiquark, or a beauty (b) quark and an antibeauty (b) antiquark. In such cases, the flavour (charm or beauty) of the quarks involved is said to be "hidden", since the quark and antiquark cancel each other out. Theorists have argued, however, that if exotic hadrons exist then states with "open", i.e. unhidden, flavour should exist. The new discovery by LHCb appears to be the first discovery of such a state.
The discovery was made through a detailed study of the beauty meson decay process B+→D+D−K+. Conventional resonances in this process can only occur in the D+D− pair, but after removing most such decays a clear peak in the D−K+ mass around 2.9 GeV/c2 is seen, as shown in the figure. The minimal quark content of a resonance causing this structure is anticharm, antistrange, up and down (csud, with the charge conjugate process resulting in csud implicitly included). The analysis of the full distribution of the sample of around 1300 B+ decays, unprecedented for this decay process in both size and low background rate, indicates that two resonances with different spins are contributing. These states, referred to as X(2900) are shown as the red and green lines in the figure, with the solid black line giving the total result of the fit which agrees well with the data points.
Prof Tim Gershon, from the University of Warwick, who worked on the analysis commented: "The LHCb data sample has rewarded us once again with this completely unexpected discovery. We are sure that these results will have theorists scratching their heads, and we are looking forward to testing the new models that are certain to be put forward. The ongoing upgrade of the LHCb detector will allow us to accumulate a much larger data sample in the next run of the Large Hadron Collider and further refine our understanding of fundamental interactions.".
More details can be found in the slides of the CERN seminar and in the forthcoming LHCb papers.
Discovery of a four-charm tetraquark?
1 July 2020
The LHCb Collaboration has today released new results which could be interpreted as the discovery of a new type of particle, called a tetraquark, made from two charm quarks and two charm antiquarks.
Thanks to tremendous attraction of the strong force, quarks are (almost) always locked up inside composite particles known as hadrons. Until relatively recently, only two types of hadrons had ever been seen in experiments; mesons made from a quark and an antiquark, and baryons made of three quarks. However, when Murray Gell-Mann and George Zweig first proposed the quark model in 1964, they speculated that four-quark and five-quark states known as tetraquarks and pentaquarks might also exist in nature.
It was not until 2014 that the first of these exotic hadrons was conclusively identified. That year, LHCb published measurements showing that the Z(4430)+ particle first observed by the Belle collaboration several years earlier, was made of two quarks and two antiquarks bound together. Then, just a year later, LHCb discovered pentaquark states made from four quarks and an antiquark. What remains in doubt is the substructrure of these exotic hadrons. Theoretical models exist for both "compact" tetraquarks and pentaquarks, in which all the quarks and antiquarks are tighly bound together, or for "molecules", in which mesons and baryons are more loosely bound together. The evidence so far has tended to support the molecule interpretation, however this latest LHCb discovery suggests compact tetraquark states may also exist.
The tetraquark candidate was found by its decay into two J/ψ mesons, each of which is made from a charm quark and charm antiquark, implying that the new particle is made from two charm quarks and two charm antiquarks. It is seen in the plot as the narrow structure peaking near 6900 MeV/c2 in the invariant mass of the pair of J/ψ mesons. This is the first time an exotic hadron has been found made exclusively of heavy quarks of the same flavour. Its properties line up nicely with what would be expected from a compact tetraquark, but the jury is still out with other interpretations also possible.
"This new result provides further crucial evidence on the behaviour of quarks and how they interact through the strong force. It will undoubtedly be of great interest to theorists working to better understand exotic hadrons." commented Prof. Tim Gershon from the University of Warwick and spokesperson for LHCb-UK. "Data that we will collect with an upgraded detector in the coming years will allow us to widen the search for further such particles, and may resolve once and for all the debate over their substructure."
More details can be found in the preprint, submitted for publication in Science Bulletin.
Understanding the puzzle of the χc1(3872) state
28 May 2020
Studies of hadron states allow us to understand the properties of the strong nuclear force that binds quarks together. Since its discovery in 2003 by the Belle collaboration there has been much speculation about the nature of the χc1(3872) state. Its properties are not consistent with it being a conventional charmonium state composed of charm and anticharm quarks. Particularly intriguing are the narrow natural width of the state and the proximity of its mass to the DD* threshold. This has led to speculation that it is more exotic in nature, e.g. that is is a four-quark state (tetraquark) or a bound DD* molecular state. Despite seventeen years of experimental study the exact nature of the χc1(3872) state remains a puzzle. New precision measurements, released today by the LHCb collaboration, shed light on this longstanding mystery.
The new results from the LHCb collaboration come from two different analyses that make precision studies of the χc1(3872) lineshape using its decay to the J/ψπ+π− final state. In the first, about 15,500 χc1(3872) particles produced in decays of hadrons containing b quarks were selected inclusively. In the second, about 4230 B+→χc1(3872)K+ exclusive decays were selected. The image shows the accumulation of events around an invariant mass of 3.872 MeV/c2 in the second data set.
In the case of perfect experimental resolution, the lineshape in this invariant mass distribution would have a peak at the mass of the decaying particle with a spread determined by the rate of its decays (according to Heisenberg's uncertainty principle, higher decay rates and hence shorter lifetimes of particles are related to larger natural widths). The functional form usually used by particle physicists to fit such distributions is called a Breit-Wigner lineshape, and one important new result is that the natural width of the χc1(3872) particle is found to be non-zero, when fitted with this model. However, it is also known that the Breit-Wigner function does not address effects on the distribution that can be caused by thresholds in invariant mass at which new decay channels become accessible. The difference of the χc1(3872) mass to the sum of the D and D* meson masses is found, in the new analyses, to be only 70±120 keV, an improvement in precision of more than a factor of two compared to previous measurements, so effects related to the DD* threshold must be taken into account in a proper description of the lineshape. To this end, a detailed study was carried out with the larger inclusive J/ψπ+π− sample, leading to important new insight that supports the hypothesis that the χc1(3872) particle contains both charm-anticharm and DD* molecular components
Dr Matthew Needham, from the University of Edinburgh, who led one of the two studies commented: "This is a breakthough in studies of the χc1(3872). The puzzle of its nature is not solved fully, but we now have a clear roadmap to fill in the last pieces. The next major challenge will be to make a simultaneous analysis of the χc1(3872) lineshape in the J/ψπ+π− and DD* decay modes. The LHCb detector is currently being upgraded to allowing an order of magnitude more data to be collected when the LHC restarts running in 2021, which will facilitate this work."
More details can be found in the preprints on the inclusive and exclusive analyses, submitted to the Physical Review D and the Journal of High Energy Physics, respectively.
Observation of new charm baryon states
2 April 2020
The LHCb collaboration has, this week, announced the observation of three new charm baryon states.
The strong interaction confines quarks into composite objects – hadrons – containing several quarks. A baryon is a type of hadron with three quarks, a well known example of which is the proton, which contains two up quarks and a down quark. The new LHCb analysis considers baryons in which one of the three quarks is a charm quark. The first such charm baryon was discovered in the 1970s but a multitude of different species have since been observed. Hadrons can be seen in various “excited” states, in analogy with the characteristic line spectra of atoms.
The new analysis searches for excited states of the Ξc0 baryon, which contains a charm quark, a strange quark and a down quark. These states, denoted Ξc**0, are short lived and rapidly decay into lighter particles, for example a Λc baryon and a negatively charged kaon. The large dataset recorded by LHCb during 2016-2018 contains several hundred thousand such decays. The figure shows the invariant mass distribution of the Λc+K− candidates, in which the new Ξc**0 states are characterised by narrow peaks sticking up above the smooth distribution of background combinations. Three new Ξc**0 states can be clearly seen in the figure. A detailed statistical analysis of the data unambiguously confirms these states with the gold standard for the claim of “observation”. The two lower mass states are observed for the first time, while further investigation is needed to understand whether the third peak is consistent with originating from a previously observed particle.
The LHCb detector is currently being upgraded so that it can restart operations in 2021 with a factor five increase in the proton-proton collision rate. The upgrade will be particularly transformative for the analysis of charm hadron decays because a new “trigger” system will permit them to be recorded with higher efficiency than the current experiment.
Emmy Gabriel, a Ph.D student from the University of Edinburgh who performed the analysis, commented “It took a lot of hard work to analyse these data so I’m really excited about the new states and how the particle physics community will interpret them”. Marco Pappagallo, a researcher at the University of Edinburgh who proposed the analysis and worked closely with Emmy, added “These results will help us to understand how quarks are bound into hadrons, but analyses of other decays are still required. It will be interesting to see if theorists can make a consistent description of these new results and others we have published previously.” (See this link for relevant previous results.)
More details can be found in the preprint that was submitted for publication in Physical Review Letters.
B0→K*0μ+μ−: new measurements confirm an old puzzle
10 March 2020
The LHCb collaboration has this week presented a long awaited analysis of the angular distribution of the rare decay >B0→K*0μ+μ− in a seminar at CERN. The new analysis uses twice the number of decays of any previous study and is of interest because of tensions with predictions of the Standard Model (SM) observed previously in this process. The new analysis confirms these tensions.
The analysis of B0→K*0μ+μ− decays is a very promising way to search for the effects of as-yet-undiscovered particles. At the quark level, this decay proceeds via a beauty- to strange-quark transition that is mediated by well-known SM particles. The process could also be mediated by new particles, which could dramatically alter the measured properties of the decay. The masses of the new particles could be well beyond those accessible directly in proton-proton collisions at the Large Hadron Collider. Previous measurements have generated some excitement in the scientific community, as the tensions observed form a coherent pattern that can be explained in various extensions of the SM that have additional fundamental particles.
The angular distribution of the particles is particularly sensitive to new effects. One quantity that can be measured from the angular distribution of the particles is denoted P5’. The measurements of this quantity are shown in the figure as a function of the invariant mass squared, q2, of the muon pair. The black points show the new results and the SM predictions are presented as orange boxes. At low values of q2, there is poor agreement between the measurement and the predictions. Effects are also seen in several other quantities measured. Taken together, the measurements are more consistent with there being a new effect than they are with the SM, although not yet significant enough to allow physicists to claim definitively that something new is being observed.
Felix Kress from Imperial College London, a PhD student who worked on the analysis, commented that “The data are very intriguing and it is exciting to think we might be seeing the first hints for a new fundamental particle. Since we started the analysis, we've recorded even more data that will enable us to study these effects in more detail in the future.” Dr. Tom Blake from the University of Warwick added “We are currently installing a major upgrade of our detector and expect to have even more data to analyse in the near future. It’ll be interesting to see what new insights those data bring.”
More details can be found in the CERN seminar, and in the LHCb paper, submitted to the journal Physical Review Letters.
Understanding CP violation in B+ → π+π+π− decays
12 September 2019
The LHCb collaboration has, this week, published long-awaited results on matter-antimatter asymmetries in B+ → π+π+π− decays. For the first time, the curious variation of the asymmetry across the phase space of the decay has been explained in terms of contributions from different resonances.
New sources of CP violation (CPV) are needed to explain the absence of antimatter in our matter-dominated universe. Until very recently, observations of CPV in B mesons had only been made in two-body decays. However, it has long been conjectured that the complex dynamics of multi-body decays could give rise to other manifestations. The B+ → π+π+π− decay proceeds mainly via various intermediate resonances — effectively, a cascade of two-body decays. The size of the phase space allows a rich tapestry of resonant structures to emerge, bringing quantum-mechanical interference into play. Much as in Young’s double-slit experiment, the total amplitude comprises the sum of all possible decay paths. The interference pattern can contribute to CPV in regions where resonances overlap.
In the new analysis, CPV effects in B+ → π+π+π− decays are described with explicit amplitude models for the first time. As shown in the figure, the models accurately follow the variation of the asymmetry between B− and B+ decays with the π+π− invariant mass. The asymmetry is also remarkably different depending on whether the cosine of the helicity angle θhel is positive (blue) or negative (red). (If, in the rest frame of the B, the pion with the same charge as the B has higher momentum than its oppositely charged counterpart then cos(θhel)>0.) The bands indicate the full spread of the three models used to describe the decays.
The new LHCb measurements are based on data from Run 1 of the LHC and contain several groundbreaking results, including the largest CP asymmetry in a single component of an amplitude analysis, found in the ππ ↔ KK re-scattering amplitude; the first observation of CPV in the interference between intermediate states, seen in the overlap between the dominant spin-1 ρ(770)0 resonance and the π+π− S-wave; and the first observation of CPV involving a spin-2 resonance of any kind, found in the decay B+ → f2(1270)π+. These results provide significant new insights into CPV.
Tom Latham from the University of Warwick, who worked on the analysis, commented "These results are the culmination of several years of effort to improve the models and account for a number of subtle effects. We now eagerly anticipate the reaction from our theory colleagues that will help us to progress further in understanding CP violation." Prof. Tim Gershon, also from Warwick and spokesperson for LHCb-UK, added "The data samples already accumulated by LHCb are providing insights into the deep issue of matter-antimatter asymmetries. It is extremely exciting to think about what we will learn from the data to be collected with our upgraded experiment, which will start operating in 2021."
More details can be found in LHCb papers, arXiv:1909.05211, submitted to PRL, and arXiv:1909:05212, submitted to PRD
LHCb physics entertains festival crowd
7 August 2019
This July, the Bluedot Festival celebrated music, art, and science for the fourth time under the Lovell telescope of the now UNESCO World Heritage Site Jodrell Bank Observatory. In the line-up of many astrophysicists and other prominent scientists, LHCb scientist Marco Gersabeck presented one of the experiment’s biggest discoveries: the first observation of charm CP violation (see article from 21 March 2019).
In a 40-minute presentation entitled "The Big Bang ABC" Marco presented a talk on Antimatter, Bananas, and Charm on the festival’s premier science stage, Mission Control. The journey into the quantum world went via the Lovell telescope, which is approximately the size of an atomic nucleus if the atom is the size of the earth. The talk then went bananas, pointing out that the fruits emit antimatter, but are completely harmless. A set of smileys was invoked to explain CP symmetry before turning to the main discovery.
The talk also highlighted the detector that’s behind the discovery, the large amount of physicists that is required to conduct such an experiment, but equally the importance of the many engineers and technicians without whom such an endeavour would be impossible. The last part of the journey went to the International Space Station where antimatter is studied as well as in PET scanners that rely on the same antimatter emission process seen in bananas. The talk was followed by 20 minutes of discussions with an enthusiastic crowd on topics ranging from music to string theory and of course the Big Bang.
Further information how antimatter is used in science are available on the Antimatter Matters website. More background about the discovery of charm CP violation can be found in an article published in The Conversation.
Updated measurements of CP violation with Bs0 mesons
17 June 2019
The LHCb collaboration has, this week, published two new measurements of CP-violation observables with decays of Bs0 mesons. These analyses encorporate LHCb Run II data from 2015 and 2016.
CP-violation is a phenomenon whereby physics is changed under the combined inversion of C(harge) and P(arity). This has long been established in strange and beauty hadron systems, and was discovered by LHCb in the charm sector earlier this year (see Observation of CP violation in charm decays). CP-violation results from subtle differences in "phases" of quantum mechanical waves. Picture two nearby stones falling into a pool of water. Their interfering wave pattern will depend on whether the stones hit the water at the same time or at different times – the difference in times corresponds to a relative phase between the respective waves. The new results concern a key type of CP violation that is yet to be observed in the Bs0 meson system. A special feature of Bs0 mesons is that before they decay (after around one picosecond on average), they "mix" between their particle and antiparticle states. A relative phase between the mixing and decay can result in a decay-time dependent CP-violation pattern. The relevant phase, denoted φs, is tiny in the Standard Model, roughly 2° (where 90° would separate a peak from a trough in our wave picture), making it highly sensitive to phases from new physics. In our water pool analogy the effect of new physics is equivalent to dropping a small third stone, subtly changing the interfering wave pattern.
The decay Bs0 → J/ψφ, where the J/ψ and φ particles are both quark-antiquark bound states (cc and ss, respectively) is considered a "golden mode" to search for this type of CP violation. The decay to two φ mesons is rarer but presents independent observables with complimentary new physics sensitivity – for example testing the Standard Model prediction that φs should be approximately the same when measured in both J/ψφ and φφ final states. Both analyses require a complicated study of how the decay rates depend on time and the angular configuration of the final-state particles. A key challenge is to determine whether a Bs0 or Bs0 was initially produced in the hadron collision. Dedicated algorithms analyse the properties of each event to determine a most-likely initial flavour (Bs0 or Bs0) and a probability of this decision being correct. Compared to previous analyses the effective performance of these algorithms has been improved by around 30%.
The Bs0 → J/ψφ analysis included roughly 120,000 decays, and yields a measurement of φs = −0.083 ± 0.041 ± 0.006 rad, which is around 2.5 standard deviations away from zero, but consistent with the precise prediction of the Standard Model, −0.0370 ± 0.001 rad. Combining with previous LHCb measurements a new LHCb average of φs = −0.041±0.025 rad is obtained. This, and the LHCb determination of the related parameter ΔΓs, are represented by the blue ellipse in the figure. The Bs0 → φφ analysis included roughly 9000 decays, and yields a measurement of φs= −0.073 ± 0.115 ± 0.027 rad, also consistent with the Standard Model predictions.
Jennifer Zonneveld and Konstantin Gizdov, both from the University of Edinburgh, are pleased with the new Bs0 → J/ψφ result. "This was a challenging and complex analysis, but it is rewarding to make significant improvements in the analysis sensitivity" says Konstantin. "It is really exciting to see how we are rapidly closing in on sensitivity to the SM value of φs. There is a strong chance that new physics may appear as we analyse future data from LHCb" says Jennifer. "The precision that we achieve with this dataset makes me really excited about the discoveries that lie in store with the LHCb upgrade" says Emmy Gabriel from the University of Edinburgh, who led the Bs0 → φφ analysis.
A larger dataset from 2017 and 2018 is currently being analysed, and the LHCb detector is in the process of being upgraded to restart operations in 2021 with a factor of five increase in the rate of proton interactions. The upgrade will be particularly transformative for decays to hadronic final states, like Bs0 → φφ, for which the hardware trigger of the current detector is a limitation.
More details can be found in LHCb papers, arXiv:1906.08356, submitted to EPJC, and arXiv:1907:10003, submitted to JHEP
New test of lepton universality in B decays
22 March 2019
The LHCb collaboration have announced an updated measurement of a quantity called RK, which is perhaps the most keenly anticipated result in particle physics. The measurement tests whether different types of leptons (electrons and muons) are produced at identical rates in B meson decays as expected in the Standard Model. The results are significantly more precise than previous measurements, and leave a tantalising hint that a new theory may be necessary to explain the data, which can be tested with improved measurements in future.
One of the great mysteries of physics is why are there so many different types of fundamental particles? Almost all the observable matter in the Universe is made up of two types of quarks (up and down) and two leptons (the electron and its associated neutrino). But there also exist two more "families" of these particles. On the discovery of the first of new particles, the muon (which is the second-family counterpart of the electron), the famous physicist Isidor Rabi commented "Who ordered that?" Physicists today are still attempting to address this question.
Although the Standard Model does not tell us why muons exist, it does make a firm prediction that all different types of leptons have identical interactions, or coupling strengths, up to effects related to their mass. This feature is referred to as lepton universality. Theories that extend the Standard Model can, however, introduce new particles that have different couplings to electrons and muons, making studies of lepton universality an excellent way to test the Standard Model.
The LHCb experiment has previously published results (see, e.g., here) that hint at a possible deviation from the Standard Model prediction of lepton universality, but at an inconclusive level of significance. Updates of these measurements have therefore been highly anticipated. The new result is a measurement of the quantity RK, which is the ratio of the rates at which a charged B meson decays into a charged kaon and either a μ+μ− or e+e− pair, and is predicted to be unity in the Standard Model.
In order to achieve the required level of precision, the LHCb measurement is made as a double ratio, in which the rates of the muon and electron modes are separately normalised to the decays to the same final state through an intermediate resonance called the J/ψ, which is composed of a charm quark-antiquark pair. Because the decays B+ → J/ψK+ → μ+μ−K+ and B+ → J/ψK+ → e+e−K+ are known to respect lepton universality, this allows effects related to the reconstruction of muons or electrons to cancel out and therefore not cause any bias on the measurement of RK.
The new result is RK = 0.846 +0.060−0.054+0.016−0.014, which is 2.5 standard deviations away from the Standard Model value of 1. The figures show the yields in the (top) K+μ+μ− and (bottom) K+e+e− final states, with fits to the data superimposed as solid lines. Also shown in dashed mauve lines are what would be predicted in the K+μ+μ− spectrum according to the observed yield of K+e+e− decays (and vice versa) assuming RK = 1.
"This measurement sets the stage for much more detailed studies of lepton universality with the LHCb detector," commented Paula Alvarez Cartelle from Imperial College London, who worked on the measurement. "We have demonstrated excellent understanding of all the subtle effects that are related to the reconstruction of electrons." Mitesh Patel, also from Imperial College London, added "The significance of the deviation from the Standard Model is not at a level of a discovery — yet. But the upgraded LHCb detector will allow us to record a hugely increased amount of data, that may well provide the sensitivity we need to establish effects from beyond the Standard Model"
More details can be found in the LHCb public webpage, and the LHCb preprint, which has been submitted for publication in the journal Physics Review Letters.
Observation of CP violation in charm decays
21 March 2019
The LHCb experiment has announced the first ever observation of CP violation in the decays of charm mesons. This represents a milestone in particle physics.
Symmetries play a crucial role in the fundamental laws of physics. Two symmetries that are of particular interest are those of the charge (C) and parity (P) transformations. Under C a particle is transformed into its antiparticle partner, e.g. an electron transforming to a positron. A system is transformed by P into its mirror image, in which all spatial coordinates are reversed, just as a left-hand appears like a right-hand when viewed in a mirror. Of the fundamental forces in nature, only the weak interaction breaks the symmetry under C and P, as discovered in 1957 by Chien-Shing Wu. But even this occurs in a such a way that the product (CP) is — mostly — still conserved.
In 1964 an experiment led by Jim Cronin and Val Fitch discovered, through studies of particles containing strange quarks, that the weak interaction violates CP symmetry. This remarkable discovery, that resulted in the award of the 1980 Nobel Prize in Physics to Cronin and Fitch, was not understood until 1973 when Makoto Kobayashi and Toshihide Maskawa proposed that the results could be explained if there were six different types of quark, rather than the three that were known at the time (up, down and strange). The charm, beauty and top quark were indeed subsequently observed in high-energy colliders in the following decades.
Kobayashi and Maskawa's theory also predicted a pattern of CP-symmetry breaking effects in particles containing the different quarks. For example, B mesons, which are particles that contain beauty quarks, were predicted to violate CP symmetry in a specific way. The confirmation of this by the BaBar and Belle experiments in 2001 led to the award of the 2008 Nobel Prize in Physics to Kobayashi and Maskawa. The theory also predicts that D mesons, which contain charm quarks, should have only very small — but non-zero — CP violation. Searches for such effects have been ongoing for several decades while becoming increasingly more precise.
The high rate and high energy of LHC collisions means that unprecedented samples of D mesons have become available, and the LHCb detector is designed to be optimal for their study. The new analysis looks at neutral charm meson decays into pairs of charged kaons (K+K−) or pions (π+π−). In these cases the particle and antiparticle versions of the charm mesons, D0 and D0, are indistinguishable from their decay products, but they can be "tagged" by how they are produced. In the figure, the peak arises from D0→K+K− decays that are produced in association with a positively charged pion. Similarly, a peak with an associated negative pion must come from D0 decays.
If CP symmetry is respected, D0 and D0 mesons will decay to K+K− at exactly the same rate, and likewise for decays to π+π−. However, it is not sufficient to measure CP asymmetries, i.e. differences between D0 and D0 decay rates, by simply counting the numbers of decays, because subtle biases may be introduced by the fact that both the beams collided by the LHC accelerator and the LHCb detector are made of matter, not antimatter. This means that D0 and D0 mesons can be produced at slightly different rates, and that the reconstruction efficiencies for particles and antiparticles may also differ. Overcoming these possible biases was the main challenge in the analysis.
A simple but clever strategy to achieve this was first developed by LHCb UK physicists at the University of Oxford. In this approach, a quantity called ΔACP, which is the difference between the CP asymmetries of the decays to K+K− and π+π−, is measured, thereby cancelling out effects of production and detection asymmetries. The new analysis includes all the data collected by LHCb during the LHC Run 1 (2011–2012) and Run II (2015–2018), yielding a result of ΔACP = (−15.4 ± 2.9) × 10−4. The central value is more than 5 standard deviations away from zero, which is the gold standard in particle physics for claiming observation of a process.
Several theoretical groups have made predictions for ΔACP in the Standard Model, giving values that are consistent with the new measurement although with relatively large uncertainties. The improved experimental data will allow theoretical predictions to be refined, so that this milestone marks the beginning of a programme of exploration. In particular, the search for CP violation in charm–anticharm mixing will provide exciting possibilities to test for sources of CP violation beyond that in the theory of Kobayashi and Maskawa.
"This is a wonderful result that demonstrates the immense capabilities of the LHCb experiment in precision studies of the charm sector", comments Eva Gersabeck of the University of Manchester, who developed many of the methods used in the analysis. "This observation opens the door to a new era of discoveries of CP violating phenomena in the charm sector with the far larger datasets expected with the LHCb upgrades." adds Mark Williams, who coordinates the working group in LHCb in which the analysis was performed.
More details can be found in the CERN press release, LHCb public webpage, and the LHCb preprint, which has been submitted for publication in the journal Physics Review Letters.
Neutral charm mesons have different masses
8 March 2019
The LHCb collaboration has submitted for publication a world-leading measurement of a process in which a neutral charm meson spontaneously changes into its anti-particle counterpart. The measurement exploits a new technique, recently proposed by members of the collaboration, and gives results that, when combined with previous measurements, show for the first time that the masses of the two neutral charm mesons differ.
The two neutral charm states denoted D0 and D0, with quark content cu and uc respectively, can "mix" through quantum mechanical processes to form the physical mesons that are observed in experiments. When the mixing proceeds via exchange of "virtual" high mass particles, potentially including as-yet undiscovered new particles, the two particles which are formed by mixing can have different masses. While such processes have been well studied for particles including the K0 (sd), B0 (bd) and Bs0 (bs) and their antiparticles, a peculiar feature of charm mesons is that their mixing rates are very small and previous experiments have not been able to establish a non-zero mass difference.
Despite its many successes, the Standard Model (SM) of particle physics is known to be an incomplete theory. One glaring problem with the SM is its inability to explain the overwhelming dominance of matter over antimatter in the Universe. For this imbalance to exist, symmetry under the combined charge-conjugation and parity (CP) operation must be violated in ways that are currently unknown. This makes it particularly interesting to study charm mixing, since if indeed as-yet unknown particles contribute they may lead to observable CP violation effects where the SM predicts CP symmetry.
Enormous numbers of charm mesons are produced in LHC collisions, and the LHCb collaboration has previously used these to make a wide range of measurements. But it had not been possible to study precisely the mixing effect since the conventional methods used to do so suffer from large uncertainties. Recently, however, several members of the collaboration proposed a new method in which subsets of the decays where either a D0 or a D0 turns into three particles (KS0π+π−) are compared against each other. Studying the dependence on decay time of the ratios of yields in subsets that are symmetric with respect to π+ ↔ π− interchange allows the identification of a D0 component in a sample produced as D0 mesons, and vice versa. Any difference in the rate between these CP-conjugate reactions would be a signal for CP violation.
The LHCb analysis is based on charm mesons collected during 2011–12. Production modes in which the charm meson is produced together with either a charged pion or muon are used, since the charge of the accompanying particle can be used to distinguish D0 from D0. The results for the charm mixing parameters are the most precise to date. When combined with previous measurements, the mass difference between the two neutral charm mesons is found, for the first time, to be significantly different from zero. This in turn leads to much better sensitivity to CP violation, since such an effect can only be determined precisely if the mass difference is non-zero. This is shown in the figure, where the allowed regions for the CP violation parameters φ and |q/p|−1 are shown before and after the new measurements are included. The no-CP violation point, as expected in the SM, is at (0,0) in this figure.
LHCb is now planning to analyse the remainder of the data sample already recorded with this new method, as well as preparing for the collection of even larger samples with an upgraded detector. "While this measurement is impressive in and of itself, the approach developed and information learned will allow us to study much larger data samples, leading to even more dramatic improvements in our knowledge", comments Dr. Nathan Jurik from the University of Oxford who worked on the analysis. "If CP violation effects are present in the charm system, they won't be able to hide much longer."
More details can be found in the LHCb preprint at arXiv:1903.03074, which has been submitted for publication in the journal PRL.
An unexpected new charmonium state
1 March 2019
The LHCb collaboration has this week annouced the discovery of a new particle made of a charm and anti-charm quark. This result is the first to make use of the full dataset accumulated by the experiment in the period 2011–2018.
Charmonium states are bound systems of a charm and anti-charm quark, held together by the strong nuclear force. Just like ordinary atoms, e.g. hydrogen, the two quarks can be arranged in various quantum states with different angular momenta and spin configurations, giving rise to a spectrum of particles with different masses. The discovery of charmonium resonances in 1974 had a large impact on the development of the Standard Model of Particle Physics. In recent years there has been renewed interest in charmonium spectroscopy following the discovery of several states that do not fit well into the expected spectrum. These new states are candidates to be exotic particles called tetraquarks that contain four quarks. To be sure that the new 'XYZ states' are really exotic it is important to find and account for all the predicted conventional charmonium states.
Charmonium states with a mass larger than twice that of the D meson, which is the lightest meson containing a single charm quark, typically decay to give a charm meson pair. LHCb has studied the decays of charmonium to neutral and charged pairs of D mesons (see the figure). Several features can be seen in the mass spectrum. The broad peaks around 3770 MeV/c2 and 3930 MeV/c2 are due to already well-studied charmonum resonances and the sharp peak to the far left due to feeddown from another known state. The surprise on this plot is the narrow peak at 3842 MeV/c2. No charmonium state with this mass has previously been observed. "The observation of this state was totally unexpected since it has such a clear signal," commented Dr Matthew Needham from the University of Edinburgh who led the analysis. "Initially, we wondered if it could be real, but then we realized there is a predicted charmonium state with that mass that had not been seen before." The observed mass and narrow natural width of the new state, dubbed the X(3842), suggest it could be the previously unobserved ψ3(1D) charmonium state. The fact this state has spin-3 makes it hard to produce, explaining why previous experiments had not seen it. Further theoretical work will be necessary to understand if its expected production rate in LHC collisions is consistent with the LHCb observations. Dr. Needham added "This discovery shows the power of the full LHCb dataset to make new discoveries. We are looking forward to further exciting new results as we analyse our current data, and in the future with even larger samples that will be collected with the upgraded LHCb detector."
The results were first presented at the PsiPhi19 conference, in slides that are publicly available. A preprint is in preparation and will be submitted for publication shortly.
News from 2018 and before
- LHCb's take on rare fully-leptonic decays
- Bright long term future for LHCb
- LHCb in fixed target mode and cosmic antiprotons
- Former LHCb Spokesperson elected Fellow of the Royal Society
- Forward tops
- Searching for CP violation in Bs meson decays
- LHCb sheds new light on dark photons
- LHCb makes a splash in Venice
- Observation of the doubly heavy baryon Ξcc++
- New LHCb results hint at possible deviations from the Standard Model
- Five new particles discovered at once
- LHCb results presented at ICHEP 2016
LHCb's take on rare fully-leptonic decays
19 December 2018
The LHCb collaboration has submitted for publication the results of an innovative new analysis, making the first measurement of the branching fraction of the rare B+ → μ+μ−μ+ν decay. This represents an important step in studies of charged B meson decays to final states containing only leptons. Modes such as B+ → μ+ν and B+ → τ+ν have been intensively searched for at previous experiments, but are difficult to study at LHCb because they contain only one charged particle in the final state. However, in the B+ → μ+μ−μ+ ν process a vertex can be formed by three muons, allowing background to be rejected by requiring displacement from the proton-proton collision point.
Fully-leptonic charged B meson decays are of interest as they are sensitive to the strength of the coupling between the b and u quarks, |Vub|, which is one of the crucial parameters of the flavour sector. This can be seen from the diagram, where the b and u quarks in the B meson annihilate to produce a W boson which then decays weakly to a muon and a neutrino. In addition, a virtual photon is radiated and decays into a pair of oppositely charged muons. A recent theoretical prediction indicates that this decay should occur only once in every 40 million charged B decays. Careful data processing must be put in place in order to filter these decays from the potentially large number of random combinations of particles from proton-proton collisions that might obscure the signal.
The analysis is based on a data sample corresponding to about 5 fb−1 of integrated luminosity collected from 2011 to 2016, and exploits the unique strengths of the LHCb detector. Excellent vertex and momentum resolution allow the flight directions of the B meson and of the three muon system to be measured. The difference between the two is used to obtain an estimate of the neutrino momentum, even though the neutrino itself does not leave any signal in the detector. This in turn enables an estimate of the B mass, known as the corrected mass, to be made. Together with the identification of muons using dedicated subdetectors, this provides powerful background rejection.
The corrected mass distribution obtained from the data is shown in the figure. A model is fitted to the data that includes components for the B+ → μ+μ−μ+ν signal as well as backgrounds due to random combinations of muons (”Combinatorial”) or from hadrons misidentified as muons (”Misidentified”). There is no evidence for the signal and hence an upper limit on the branching fraction of around 1 in 60 million decays is set at a confidence level of 95%. To provide a feeling for how the spectrum would have looked like if the signal was present, the figure also includes a line showing the expectation corresponding to the recent theoretical prediction. The intriguing disagreement between the prediction and the data is expected to stimulate further theoretical investigations of this mode. Slavomira Stefkova from Imperial College London, who led the analysis, commented "Thanks to the impressive capabilities of the LHCb experiment we have not only reached but surpassed the sensitivity at which it was predicted we should see this rare decay. This leaves us in a puzzling situation that we hope to understand through progress in both theory and experiment."
The LHC Run II, which has recently concluded, has led to a doubling in the amount of recorded data compared to the current analysis. In the next two years the LHCb detector will be upgraded to allow further significant increases in the data sample. This provides excellent potential for further improvement in the searches for this and related rare B meson decay modes. Lucia Grillo from the University of Manchester, who convenes the working group in which the study was carried out, said "This analysis highlights the exciting prospects for future LHCb upgrades."
More details can be found in the LHCb preprint at arXiv:1812.06004, which has been submitted for publication in the journal EPJC.
Bright long term future for LHCb
28 August 2018
The LHCb Collaboration has today published a 167 page report on the physics that can be achieved with an upgrade of its detector that will allow it to continue operating as long as the Large Hadron Collider is colliding protons. Referred to as "Upgrade II" of the LHCb detector, it will allow the full exploitation of the flavour physics potential of the high luminosity period of LHC operation, in line with a key recommendation of the European Strategy for Particle Physics.
The original LHCb detector was designed to collect data delivered during about the first six years of LHC operation. This mission will be completed later this year, after which the Upgrade I detector will be installed during a two year shutdown of the LHC. The Upgrade I detector is intended to increase the current dataset by roughly a factor of six, during the period 2021-2029. The new document details the physics reach of the Upgrade II detector which will operate from 2031 onwards, allowing another factor 6 increase in the data sample as seen in the figure.
A key focus of LHCb is the study of rare decays of beauty hadrons, because their properties are highly sensitive to new physics (NP) beyond the Standard Model (SM). For example, a great success of the current experiment was the observation of the decay Bs→μ+μ− with a branching ratio of a few × 10−9. The LHCb Upgrade I detector with its new trigger system, upgraded subdetectors and increased dataset size will greatly enhance our NP sensitivity, but the precision study of even rarer B decays, for example Bd→μ+μ− (with a predicted branching ratio of around 10−10), will remain out of reach. The LHCb Upgrade II is therefore needed to get the maximum physics return on the high luminosity upgrade of the LHC machine, which will start operating around 2026 and run until the late 2030s.
An Expression of Interest in the LHCb Upgrade II was submitted in February 2017. The EOI described the detector challenges and solutions but did not fully explore the physics potential. The new document describes the broad programme that is foreseen for LHCb Upgrade II, including topics such as matter-antimatter asymmetry phenomena, ultra rare beauty decays, charm hadrons, electroweak and Higgs physics, and new exotic hadrons. Many of the measurements that will be made in these areas will be unique to LHCb, while for others the LHCb Upgrade II sensitivities surpass those of any other experiment envisaged on the same timescale.
The collaboration now intends to move forward with the necessary research and development to address the technological challenges presented by operating a precision detector in an ultra-high-rate environment. "Developing the physics case for the LHCb Upgrade II has really brought home how important detector development will be," Prof. Tim Gershon from the University of Warwick and spokesperson for LHCb-UK commented. "It is clear that we need to use fast timing to separate particles from different proton-proton collisions in each LHC bunch crossing, that will otherwise swamp signals from rare processes. This provides exciting opportunities for collaboration with industry."
More details can be found in the report, which has been made available on the arXiv preprint server at arXiv:1808.08865. This complements the Expression of Interest, which can be downloaded from the CERN document server.
LHCb in fixed target mode and cosmic antiprotons
21 August 2018
The LHCb Collaboration has submitted for publication a novel, and first ever, measurement of antiproton production in proton-helium collisions. The analysis exploits a unique “fixed-target” mode of the LHCb experiment and LHCb’s charged particle identification capabilities. The results will help us to interpret indirect searches for dark matter through the rates of cosmic antiprotons.
One of the greatest puzzles in physics today concerns the matter content of the Universe, of which ordinary “baryonic” matter only accounts for a few percent of the total. Dark matter (DM) is hypothesised as a remedy but it has not yet been directly observed. LHC experiments search for DM production in proton-proton collisions (e.g., see "LHCb sheds new light on dark photons"), while various underground experiments hunt for nuclear recoils caused by scattering with DM particles. There are also searches for signals from the annihilation in space of DM particles, which could lead to particle-antiparticle pair production. Since the natural abundance of antiparticles (e.g. positrons and antiprotons) is small, DM annihilation could lead to a significant enhancement of the antiparticle rates. The space-borne AMS-02 and PAMELA experiments have measured the ratio of antiproton and proton rates, as can be seen in the top figure. The trouble is that the predictions of the null-hypothesis are subject to large uncertainties, as represented by the coloured bands, in the rate of antiprotons produced in the interaction of protons with the interstellar medium. A particular weakness is the poor knowledge of the cross-section for the process pHe → pX. This is where this new LHCb result enters the game.
The LHC is primarily a proton-proton collider, but LHCb experiment has a unique capability in fixed target mode, thanks to its SMOG system. This allows the injection of noble gases into the interaction region. Designed to measure the LHC beam profile by imaging the interactions of the beam protons with the gas nuclei, it can also be used to study other properties of those interactions. It is straightforward to count the rate of negatively charged particles in the pHe collisions, but these are a mixture of π− and K− mesons as well as antiprotons. This is where the UK led RICH detectors are crucial. They contain gas mixtures in which light travels slightly slower than it would do in a vacuum, so that high-energy particles entering these detectors slow down by emitting particles of light. This Cherenkov light (named after the Russian physicist Pavel Cherenkov) is like the wake of boat travelling on water – the faster the boat the narrower the wake pattern. The key idea is that for the same charged particle momentum (measured by the LHCb tracking detectors), antiprotons will travel slightly slower than pions and kaons because they are heavier. The middle figure shows how information from the RICH detectors can be used to separate pions (lower central) and kaons (upper left) from antiprotons (upper right).
The new analysis uses the RICH information to separate out the antiproton component. In data recorded during a special run taken in May 2016 around 34 million pHe collisions were recorded, a yield of 1.5 million antiprotons is obtained. The antiproton production cross-section is measured in bins of momentum and transverse momentum (perpendicular to the beam direction), since different theoretical models lead to different predictions for these spectra. As can be seen in the bottom figure, the measurement does not agree perfectly with any of the predictions, suggesting that improvements in theoretical modelling of these processes is needed. By providing a means to test the theoretical models, this new measurement will greatly improve our ability to interpret cosmic antiproton rates as possible dark matter signals.
Antonis Papanestis from the Rutherford Appleton Laboratory, who is responsible for the LHCb Cherenkov detectors, said "This result demonstrates the unique capability of LHCb and the broad physics programme our detectors are able to support. We are now working to upgrade the detectors so that we can maintain this exceptional performance while collecting data at higher rates."
More details can be found in the LHCb preprint at arXiv:1808.06127, which has been submitted for publication in the journal Physical Review Letters.
Former LHCb Spokesperson elected Fellow of the Royal Society
9 May 2018
Guy Wilkinson, a Professor at the University of Oxford and a member of the LHCb UK community, has been elected as a Fellow of the Royal Society. Guy is one of 50 eminent UK scientists, and 10 from overseas, in the 2018 intake of new fellows. Others include Fabiola Gianotti, the Director General of CERN and Elon Musk, the entrepreneur behind Tesla and SpaceX.
Guy is a founder member of the LHCb collaboration, which he led as Spokesperson from 2014 to 2017 having previously been Physics Co-ordinator from 2011 to 2012. In addition to these important management positions, he is highly active in studies of CP violation in both beauty and charm systems, and in developing particle detectors to allow improved measurements in future.
Forward tops
14 March 2018
The LHCb collaboration have recently announced a measurement of the production of top-quark pairs, using data recorded in Run 2 of the CERN LHC. Top quarks are the heaviest known fundamental particle with a mass of approximately 173 GeV/c2, roughly equivalent to that of an entire atom of gold. Comparing precise measurements of the production and decay properties of top quarks with theoretical predictions gives a sensitive probe of the Standard Model of particle physics.
The study of top quarks forms a core part of the physics programme of the ATLAS and CMS collaborations, but is an area where the LHCb experiment has only recently started to contribute. However, LHCb results can provide unique insight. The LHC is a proton collider, but the proton is not a fundamental particle so at high energies the interaction in the collision is actually between the components (or "partons") of the proton, specifically quarks and gluons. At the LHC, top quarks are predominantly produced in gluon-gluon interactions. Measurements of the rate of top quark production therefore allow physicists to constrain the shape of the gluon parton density function (PDF), i.e. the amount that gluons contribute to the total constituents of the proton as a function of the fraction of the proton’s momentum carried by the parton (denoted x).
LHCb’s forward-geometry allows measurements of top quark production to be made at higher values of x than are possible with the ATLAS and CMS detectors. The theoretical uncertainties on the gluon PDF are largest at these x values, and therefore the LHCb results have a strong impact in reducing these uncertainties. This benefit comes at a price, however, as the production cross-section of top-quarks is lower at LHCb, leading to smaller data samples. Moreover, the geometrical acceptance of LHCb is such that the likelihood of all decay products being reconstructed is also lower.
Top quarks decay instantaneously after being produced, most commonly to a W boson and a b quark, with the W boson subsequently decaying to other quarks or to leptons and neutrinos. The new LHCb result searches for pairs of top-quarks decaying to a final state containing an electron, a muon and a b quark, making use of the good b-jet tagging performance of the detector. While not the most frequent of decay signatures, the increased cross-section expected in 13 TeV collisions compared to that in Run 1 makes this final state accessible for the first time at LHCb. In addition, this signature has only a small contamination from QCD background processes, leading to a pure sample of data to be analysed. The figure shows the invariant mass of the μeb combinations in the data, with the coloured histograms indicating the expected distributions of different processes, including the dominant tt component. Using this fit to the data to estimate the number of top-quark pairs, LHCb physicists measured the cross-section for tt production from pp collisions at 13 TeV, finding it to be 126 ± 19 ± 16 ± 5 fb, where the first, second and third uncertainties are statistical, systematic and due to the estimate of the luminosity used to make the measurement, respectively. This is consistent with predictions for this quantity using the latest theoretical methods.
Dr Will Barter from University of Manchester, who was a proponent of the analysis, states that “The LHCb detector gives a unique environment for studying top-quarks. The forward geometry, excellent vertex resolution and b-jet tagging typically give very pure samples for analysis in a kinematic region that is complementary to the larger ATLAS and CMS experiments.”.
Dr Stephen Farry from University of Liverpool, who presented a CERN seminar on the new result, adds “This result bodes well for future top-quark measurements at LHCb. Future upgrades to the experiment for Run 3 and beyond will further enhance our capabilities as we move towards and into the high luminosity LHC programme, leading us to the era of precision top-quark results in the forward region.”.
More details can be found in the LHCb preprint at arXiv:1803.05188, and in the CERN seminar.
Searching for CP violation in Bs meson decays
12 January 2018
The LHCb collaboration has recently published the first measurement of the CP-violating phase φsdd using the decays of Bs0 and Bs0 mesons into the K+π−K−π+ final state. This is a significant milestone in CP violation studies, and indicates exciting prospects for more precise measurements with the larger data samples that will be accumulated in future.
At quark-level, the Bs0 → K+π−K−π+ decay is a loop-mediated b → dds transition, which distinguishes the measurement from previous LHCb analyses using Bs0 → J/ψφ and Bs0 → φφ decays that are sensitive to the CP-violating phases φscc and φsss, respectively. All of these phases are generated via the interference between Bs0-Bs0 meson mixing and decay to the particular final state under study. Importantly, the Standard Model (SM) of particle physics precisely predicts that these phases should be close to zero, making them an interesting place to look for signs of beyond-the-SM physics that could introduce new CP-violating contributions.
These CP-violating phases are measured by analysing the asymmetry between the number of Bs0 and Bs0 candidates as a function of the Bs0 decay time. This requires the use of so-called flavour-tagging algorithms, which analyse the properties of each recorded pp collision in the LHCb detector to give a decision (and probability for being incorrect) about whether the preduced B meson contained a b or a b quark. Both the tagging algorithms and detector-induced decay-time resolution and efficiency effects need to be carefully calibrated using a combination of data control samples and simulation in order to maximise the sensitivity to measuring φsdd.
The invariant mass of the selected K+π−K−π+ candidates is shown in the figure, with the signal Bs0 component clearly visible as the dark green peak. A statistical treatment is subsequently used to remove the other “background” components in this distribution so that the signal part can be studied in more detail.
Previous theoretical studies of this channnel have focussed on the Bs0 → K*(892)0K*(892)0 channel, where K*(892)0 is a spin-1 resonance that decays to Kπ. LHCb researchers discovered that the sensitivity to φsdd could be improved by including also other Kπ resonances with spin-0 and spin-2. This, however, makes the analysis extremely complicated – the complete model requires a summation over 190 terms accounting for quantum mechanical interference between all the different Kπ components. In order to make the measurement, a custom-built GPU-based fitting framework was developed to drastically reduce the amount of time needed to perform all necessary computations. Using the full data sample collected during Run 1 of the LHC, the final result for the CP-violating phase is φsdd = −0.10 ± 0.13 ± 0.14 rad, where the first uncertainty is statistical and the second is systematic. This is consistent with the SM expectation and with previous measurements of φscc and φsss made by the LHCb collaboration.
Dr. Matthew Kenzie from the University of Cambridge, who worked on the measurement, states "This result is incredibly important for underpinning our understanding of CP-violation in the quark sector and helps us to provide a handle on New Physics contributions. Furthermore, it has allowed us to gain significant understanding into the Bs0 → K+π−K−π+ resonant structure. Decays of this nature are very complex to analyse and it’s fantastic that we can perform precision studies in such difficult environments."
Dr. Greig Cowan from the University of Edinburgh, who chaired the LHCb-internal review committee for the analysis, adds "This is a beautiful result, highlighting the excellent performance of the LHCb detector in vertex resolution, particle identification and tagging performance to make precision measurements of CP-violation in b-mesons."
The LHC is well into its Run 2 operations that will continue until the end of 2018, allowing LHCb to approximately double its existing data sample. An upgraded LHCb detector will then be installed, ready to begin collecting data in 2021. The larger data samples that will allow even more precise measurements to be made, providing stringent tests of the Standard Model of particle physics.
More details can be found in the LHCb preprint at arXiv:1712.08683
LHCb sheds new light on dark photons
9 October 2017
The LHCb collaboration has recently announced the results of a search for a hypothesised new particle called a dark photon. In the Standard Model (SM), photons are the quanta of light that mediate the electromagnetic force. The existence of dark matter that interacts very weakly with SM particles has led to theories about a "dark sector", with its own particles and forces including a dark photon. For these theories to be able to explain the distribution of dark matter observed in galaxies, there must be a small but non-zero coupling between the dark photon and the SM photon, which is described by a parameter labelled ε.
Several experiments have searched for dark photons over the past thirty years, without observing a signal. However, the limits obtained do not rule out many of the most interesting values of ε and the dark photon mass, motivating several proposals for new experiments. Recently researchers on the LHCb experiment realised that its precise vertex detection capability allows excellent sensitivity to dark photons to be achieved, so that significant improvement over previous results can be obtained without the need to build a new experiment.
In the new result, LHCb data is used to search for a dark photon decaying to a pair of oppositely-charged muons. The muons may originate either directly from the LHC proton-proton collision vertex (referred to as "prompt"), or may be displaced from it. The latter case is particularly interesting when the parameter ε is very small, as this causes the dark photon to travel an observable distance before decaying. The background from pairs of muons produced from interactions of SM photons in the LHCb detector material can be removed using a precise map of the detector, shown in the top figure, that was obtained as a spin-off of the analysis.
The new results using the prompt sample limit ε2 to be below a value that depends on the dark photon mass, as can be seen in the bottom figure (the new results are shown by the blue regions, along with the limits from previous experiments in grey). The new results are the most stringent constraints to date for masses between 10.6 and 70 GeV. The displaced sample allows values of ε2 of around 10−9 to be excluded for low mass dark photons, as also shown in the figure.
Dr. Phil Ilten from the University of Birmingham, who worked on the analysis, said "It's exciting that we can use the LHCb detector to test dark photon models -- this was never foreseen when the experiment was designed."
The LHC is well into its Run 2 operations that will continue until the end of 2018, allowing LHCb to approximately double its existing data sample. An upgraded LHCb detector will then be installed, ready to begin collecting data in 2021. Dr. Ilten adds "The LHCb upgrade will allow a big improvement in sensitivity, so that we will either observe dark photons, or rule out most of the theoretically favoured parameter space."
Details of the new result can be found in the preprint, LHCb-PAPER-2017-038 (arXiv:1710.02867), submitted to Physical Review Letters.
LHCb makes a splash in Venice
14 July 2017
The LHCb collaboration has released a host of new results at the EPS-HEPP conference in Venice this week. The conference is a biennial meeting of the high energy physics community, typically attracting around 1000 participants to discuss all aspects of the world-wide particle physics programme.
One highlight was the announcement of the discovery of the doubly-charmed Ξcc++ baryon (see the news item of 6 July) but many others were reported by a total of 32 speakers from LHCb covering a broad range of topics, including CP violation, rare b-hadron decays and heavy-ion physics. In particular, the collaboration announced an updated measurement of the CKM angle γ from a combination of results from several analyses of B→DK and related decays. The measurement of γ is one of the main aims of the LHCb experiment and a standard candle test for the Standard Model (SM) due to its very precise theoretical prediction. The new result gives a value of γ of (76.8+5.1−5.7)°, which is the most precise measurement to date.
A crucial aspect of measuring γ is the need to combine information from many different decay modes, as there is no single channel that dominates the precision. The LHCb collaboration is ensuring that the full potential of the dataset is exploited by making use of as many different decay modes as possible. This is highlighted by a new measurement of B→D*K decays using a novel partial reconstruction technique in which the D* → Dπ0 and D* → Dγ decays can be separated without the need to reconstruct the neutral pion or photon. The method is illustrated in the top figure, with the partially reconstructed component clearly visible to the left of the large peak from B→DK decays. The B→D*K decays with D* → Dπ0 and D* → Dγ are shown as the dark blue double humped structure and the light blue broad shape, respectively; several background decays are also shown in different colours. Donal Hill from the University of Oxford, who led the analysis, said "This is a major breakthrough for LHCb. The fact that we can use partial reconstruction to measure B→D*K decays significantly increases the range of channels that can be studied and will help us to further reduce the uncertainty on γ."
Another exciting new result is the first observation of a B0 meson decaying to a proton and an antiproton, shown in the bottom figure. Only about one in ten million B0 mesons decay in this way, making it the rarest decay mode of the B0 meson ever observed. Lars Eklund from the University of Glasgow, who worked on the analysis, said "The exquisite performance of the LHCb detector allows us to break new frontiers in sensitivity to rare decays. The ability to distinguish protons from other charged particles, provided by our ring-imaging Cherenkov counters, and the precise vertex information provided by the silicon vertex locator were both essential to this discovery."
The LHC has just resumed operations for 2017 and will continue until the end of 2018, allowing LHCb to approximately double its existing data sample. An upgraded LHCb detector will then be installed and will start collecting data in 2021, leading to significant further increases. The larger data samples that will be accumulated will enable even more precise measurements that will provide stringent tests of the Standard Model of particle physics.
Observation of the doubly heavy baryon Ξcc++
6 July 2017
The LHCb collaboration has announced the discovery of a new particle, the Ξcc++ state. Just like the protons that circulate in the Large Hadron Collider, the new particle is a baryon, composed of three quarks bound together by the strong force. However, unlike the proton which is made from three light quarks (two up quarks and a down quark), the Ξcc++ contains one up quark and two charm quarks. Since the charm quarks are much heavier than up or down quarks, the new particle has a mass more than 3.5 times larger than the proton.
Although new bound states of quarks are discovered at the rate of (typically) a few per year, this observation is particularly noteworthy as it is the first time that a baryon containing two "heavy" quarks has been seen. This opens new possibilities to investigate the strong nuclear force, which is described by Quantum ChromoDynamics (QCD) within the Standard Model of Particle Physics. In a doubly heavy baryon, the two heavy quarks are almost static at the centre of the baryon with the lighter quark orbiting around them. This is in some respects analagous to the hydrogen atom, where the electron orbits around the nucleus due to the electromagnetic force (or more precisely, due to Quantum ElectroDynamics, QED).
Doubly heavy baryons have never previously been seen, despite numerous searches. In 2002, the SELEX collaboration at Fermilab in the USA claimed to have observed the singly charged Ξcc+ state (composed of one down and two charm quarks). However, this result was not confirmed by other experiments leading to a long-lasting mystery. Using large and very pure samples of Λc+ baryons combined with negative kaons and two pions (Λc+K−π+π+) the LHCb collaboration observes a highly significant signal (> 12σ), as shown in the figure, that is identified as the Ξcc++ baryon. While the doubly-charged and singly-charged states are expected to be "partners", the properties of the observed state are not consistent with those expected based on the SELEX results.
The results were presented at the EPS HEPP conference in Venice, Italy, by Dr. Patrick Spradlin from the University of Glasgow, the lead researcher of this analysis. "The new result from LHCb does not yet resolve the puzzle raised by SELEX but it is a crucial step into an empirical understanding of the nature and properties of doubly heavy baryons" said Dr. Spradlin.
LHCb physicists are now planning a set of future studies to search for other members of the baryon family, including the singly charged Ξcc+ state. With more data LHCb will also be able to measure more properties of these Ξcc particles including their lifetimes and rates of production at the LHC.
"This spectacular result demonstrates again the superb performance of the LHCb detector" said Prof. Tim Gershon from the University of Warwick and spokesperson for LHCb-UK. "We can now be confident that with the large increases in data sample we will accumulate with an upgraded detector, we will be able to make further discoveries of doubly heavy baryons, which may lead to a real breakthrough in our understanding of QCD and quark confinement."
The measurements were made with the data taken at the LHC during 2012 and 2016. The LHC has recently resumed operations for 2017 and will continue until the end of 2018. The LHCb detector will be upgraded to during 2019-2020 such that it is ready for the next phase of the LHC, starting in 2021.
New LHCb results hint at possible deviations from the Standard Model
18 April 2017
The LHCb collaboration have just announced a new measurement that is causing excitement among particle physicists. The measurement is of a quantity called RK*, which tells us how often B0 mesons decay to a K* meson and two oppositely-charged muons relative to the case where they decay to a K* and two oppositely-charged electrons. Within the Standard Model (SM) the equivalence ('universality') of lepton interaction strengths means that RK* should be almost exactly equal to one. However, the presence of non-SM particles could modify the rate of one of these decays over the other, leading to the breakdown of lepton universality.
The LHCb collaboration has used its Run 1 dataset (from 2011 and 2012) to make the most precise measurement of RK* [1] in two different regions of q2 (the squared-invariant mass of the two lepton system) that show a discrepancy with the concept of lepton universality. The measured values are each around 2.5 standard deviations below the SM predictions as seen in the figure. Previous, less precise, measurements of RK* have been performed by the Belle and BaBar collaborations [2,3] that were consistent with predictions and the new LHCb results.
Although the significance is below the five-sigma threshold usually required in particle physics to claim a discovery, this new information goes in the same direction as a previous indication of lepton non-universality in a similar ratio, RK, which the LHCb collaboration published in 2014 [4]. Together with other measurements related to similar b hadron decays, these measurements could be explained by the existence of non-SM processes.
Dr. Simone Bifani from the University of Birmingham presented the new results in a seminar at CERN on April 18th. He says "The measurements represent a milestone for the LHCb collaboration. We have achieved excellent understanding of potential biases in the results through detailed studies of control samples. When we update the analysis to include data recorded during Run 2 we have the potential to make the first observation of physics beyond the Standard Model at the LHC."
Prof. Tim Gershon from the University of Warwick and spokesperson for LHCb-UK adds "The mood is one of cautious excitement -- no-one is popping any champagne corks yet. Detailed understanding of these deviations requires a long-term programme of measurements that we are now planning. Work is ongoing towards LHCb detector upgrades that will enable the increased sensitivity that is required."
[1] LHCb collaboration, R. Aaij et al., LHCb-PAPER-2017-013
[2] Belle collaboration, J.-T. Wei et al., Phys. Rev. Lett. 103 (2009) 171801
[3] BaBar collaboration, J. P. Lees et al., Phys. Rev. D86 (2012) 032012
[4] LHCb collaboration, R. Aaij et al., Phys. Rev. Lett. 113 (2014) 151601
Five new particles discovered at once
21 March 2017
This week the LHCb collaboration announced the discovery of five new particles known as excited Ωc (Omega_c) baryons. Baryons are composed of three fundamental particles called quarks. Well-known examples of baryons are the protons and neutrons that are found in atomic nuclei. A proton is made of two "up" quarks and one "down" quark, bound together via the strong nuclear force. The Ωc baryons are similar, but they are made from two "strange" quarks and one "charm" quark. These are like heavier (i.e., more massive) versions of the up and down quarks. The Ωc baryons do not exist inside atomic nuclei and can only be produced on earth in certain particle physics experiments such as the CERN Large Hadron Collider.
The first Ωc baryon (the ground-state) was studied over 20-30 years ago. By giving energy to the quarks inside the baryon, it is possible to "excite" the baryon into a new state, somewhat like the way that atoms can be excited into a new state. These states typically have different orbital angular momentum between the constituent quarks and the baryon is more massive. This is what we see in the data, where we have five very distinct peaks at five different values of the mass from 3 GeV up to about 3.1 GeV. For comparison, the proton has a mass of about 0.9 GeV (GeV is a convenient unit used for measuring particle masses).
These five new states were expected to exist, but had never been seen before. It is only with the CERN LHC that we have the ability to produce these states in large numbers, which can then be detected through the excellent performance of the LHCb detector. The next steps will be for LHCb collaborators to try to measure the quantum numbers of these states to see if they match up with theoretical predictions that are based on the theory of the strong force (Quantum Chromodynamics - QCD). This should be able to confirm how we think QCD operates or lead to refinement of the theorists predictions.
This discovery shows the amazing potential of the LHCb experiment to further understand QCD. It will help theorists better understand how quarks and gluons bind together into baryons and, in particular, how the spin correlations between the constituent quarks play a role in that binding. This will have interesting implications as we search for more exotic multi-quark states such as pentaquarks and tetraquarks using data from LHCb.
Dr. Greig Cowan, LHCb physicist at the University of Edinburgh says "What is fantastic is that this observation was performed with only a subset of the currently available data. We have already recorded more and will continue to take data until the end of 2018 (LHC Run 2), meaning that there could be many more surprises waiting to be discovered."
Prof. Tim Gershon, spokesperson for the LHCb-UK collaboration adds "Between 2019-2021 we will upgrade the LHCb detector, ready to start again for LHC Run 3. I anticipate that there will be much more to learn about the strong nuclear force once we get our hands on that data!"
LHCb results presented at ICHEP 2016
3rd August 2016
This week sees the start of the big summer event in particle physics: the International Conference on High Energy Physics (ICHEP). This year it is hosted by the University of Chicago and many LHCb collaborators are now heading west to present the latest results from the experiment.
One of the highlights is the first measurement of the photon polarisation in radiative B decays. This is a crucial measurement as the Standard Model (SM) of particle physics predicts that photons are predominantly left-handed, while many beyond-the-SM models predict enhanced amounts of the right-handed component. Handedness refers to how the photon's spin rotates about its direction of motion in either a clockwise or anticlockwise direction, referred to as right- or left-handed polarisation, respectively. The LHCb collaboration have used the full Run-1 data sample of Bs0 → φγ decays to measure a quantity called AΔ, which is sensitive to the polarisation and is predicted to be AΔSM = 0.047+0.029−0.025 in the SM. LHCb has made the first measurement (LHCb-PAPER-2016-034) of this quantity to be AΔ = −0.98+0.46−0.52+0.23−0.20, which is compatible with the prediction.
Dr Tom Blake, Royal Society University research fellow and convenor of the working group that produced this results states that "This is a major milestone for us. In 2009 we set out a roadmap for six important measurements we wanted to make with the LHCb detector. This is the last measurement to be ticked off that list. With Run-2 data fast coming in we are looking forward to making even more precise tests of the photon polarisation predicted by the Standard Model."
Another new result is one of the first to use data recorded during in 2015 at the start of Run-2 of the LHC. With that dataset LHCb has measured (LHCb-PAPER-2016-031) the b quark production cross-section, which tells us how often b-quark flavoured hadrons are produced at in the 13 TeV proton-proton collisions at the LHC. The measured value is 164.9 ± 2.3 ± 14.6 micro-barns, which means that for every inverse micro-barn of data collected by the LHCb experiment in Run-2, approximately 165 b-hadrons are produced within the LHCb detector acceptance. Given that LHCb has already collected over 1 inverse femtobarn of 13 TeV collisions, this means that the experiment has recorded more than 165 billion b-hadrons! This large sample will allow LHCb to make even more precise measurements of b-quark properties in the future.
Dr Greig Cowan, STFC Ernest Rutherford fellow, helped produced the cross-section result states that "This result is a benchmark for the LHCb experiment that will be used to constrain and influence theoretical models that predict the properties of b-quark production. By measuring this basic quantity we have shown that the LHCb detector is fully operational in Run-2 and can look forward to a host of new measurements in the coming months."
LHCb will present new results from over 20 publications and conference reports. These include observations of extremely rare B meson decay modes, new constraints on CP violation effects in both charm and beauty sectors and first results with LHCb's new HERSCHEL subdetector.